Analyzing brain cell dynamics with 2-photon microscopy
Two-photon microscopy is a cutting-edge technique that allows precise, high-resolution imaging of living brain tissues while minimizing phototoxicity and photobleaching. Dr. Federico N. Soria expertly applies this method, demonstrating its transformative impact on neuropharmacological research and drug discovery.
This video is licensed under a Creative Commons Attribution-NonCommercial-NoDerivatives (CC BY-NC-ND) license. You are free to share it for non-commercial purposes with proper attribution, but no modifications or adaptations are allowed.
1. Why use this method?
Two-photon microscopy is a powerful fluorescence imaging technique that enables deep tissue visualization with high spatial resolution while minimizing phototoxicity and photobleaching. Unlike traditional one-photon (confocal) microscopy, two-photon excitation occurs only at the focal plane, providing intrinsic optical sectioning without the need for a pinhole. This makes it particularly advantageous for imaging living tissues, such as brain slices or whole organisms, up to several hundred micrometers deep. The method leverages the simultaneous absorption of two photons of longer wavelength (typically near-infrared), which enhances tissue penetration and reduces scattering, thereby allowing for detailed imaging of complex biological structures in their native environment.
2. What you’ll need
Materials and Equipment
- Two-photon microscope system:
- Femtosecond laser: titanium-sapphire (Ti) laser capable of delivering ultrafast pulses in the 690-1040 nm range or fiber-based laser (Yb).Scanning mirrors: galvanometer (galvo) mirrors or resonant scanners for beam scanning.Objective lens: high numerical aperture (NA) objectives (e.g., 20x, 40x, 60x, NA ≥ 1.0) suitable for two-photon microscopy.Detectors: photo-multiplier tubes (PMT) optimized for the emission wavelengths of the fluorophores used (made from gallium, arsenide, and phosphide).Emission filters and dichroic mirrors: to separate excitation and emission light paths based on wavelength.Sample stage: motorized stages for precise sample positioning and z-scanning capabilities.
- Cooling Systems: for both the laser and detectors, to maintain optimal performance (water-cooling for Ti:saphire laser; air-cooling for fiber-based laser).
- Sample Preparation Materials:
- Biological samples: Live specimens, appropriately labeled with fluorescent markers (e.g., acute brain slices from P20-P30 mouse with fluorescent microglia – CX3CR1GFP/+ or fms-GFP or CX3CR1Cre/Ai9). AAV-based fluorescent reporters such as GCaMP6 (for Ca2+ imaging), or permeable fluorophores such as Fluo-4 are also suitable.
- Physiological solutions: For maintaining live samples during imaging (e.g., buffered saline solutions, aCSF
- Reagents:
- Fluorophores: small fluorescent dyes or proteins: e.g., Alexa Fluor, TRITC, Fluo-4, GFP, DsRed).
- Computing Resources:
- Image analysis software: For data acquisition, control of microscope parameters, and image processing (e.g., MATLAB, ImageJ, FIJI, Python).
- High-performance computer: Capable of handling large datasets and real-time processing requirements. Preferably with a large RAM.
3. Step-by-step instructions
Step 1. Sample preparation:
- Dissect the brain from the experimental animal and quickly place it in ice-cold, oxygenated aCSF sucrose.
- Using a vibratome, cut the brain, submerged into oxygenated ice-cold aCSF sucrose, into thin slices (typically 300-400 µm thick) to ensure sufficient penetration of the laser light.
- Transfer slices to a slice keeper and maintain them in oxygenated aCSF regular at 34°C until ready for imaging.
Step 2. Microscope Configuration:
- Power on the two-photon microscope and allow the system to stabilize, particularly the Ti::Sapphire laser.
- Adjust the laser to the appropriate wavelength based on the fluorophore’s excitation peak (e.g., 920 nm for GCaMP6f, 1040 nm for TdTomato, 850 nm for Alexa Fluor).
- Calibrate the PMTs to the correct settings, ensuring they are sensitive enough to detect weak signals without being overwhelmed by noise.
- Position the sample on the motorized stage, with warm aCSF perfused at 1 mL/min (live sample).
Step 3. Imaging Procedure:
- Begin with low laser power (around 10-15 mW) to minimize potential photodamage. Adjust as necessary based on tissue depth and signal intensity.
- Acquire a Z-stack of images by incrementally moving the stage in the Z-axis (e.g., 2 µm steps), covering the desired depth of the tissue.
- Continuously monitor the live image to check for any drift or photobleaching and adjust acquisition parameters if necessary.
Step 4. Post-Processing:
- After data acquisition, process the images using software like ImageJ or Fiji. Common steps include drift correction, noise reduction, and fluorescence intensity normalization.
4. Practical tips
- Fluorophore Selection: Choose fluorophores with high two-photon cross-sections at the chosen excitation wavelength to maximize signal and minimize required laser power.
- Laser Power management: While two-photon microscopy reduces photodamage compared to one-photon methods, excessive laser power can still harm biological samples. Always start with the lowest possible laser power to minimize photodamage and photobleaching.
- Environmental control: For live imaging, maintain environmental conditions (e.g., temperature, CO₂ levels) to keep biological samples viable during imaging sessions. Moreover, keep the imaging chamber at a stable temperature and free from vibrations to avoid artifacts in the images.
5. Critical appraisal & implications for future research
Two-photon microscopy offers high benefits for in-depth imaging of live tissues, particularly in neurobiological research. Its ability to image living cells with high resolution and minimal photodamage is unmatched by other optical methods. However, the high cost and technical complexity of the equipment can be limiting. Future research should aim to simplify the operation of two-photon systems and reduce their cost. Moreover, advancements in fluorophore design could enhance the specificity and brightness of the signals, allowing for even deeper tissue penetration and clearer imaging. Enhanced software tools for real-time data analysis and artifact correction could also expand the applicability of two-photon microscopy, making it accessible to a broader range of research laboratories.
This protocol is part of the Braining project, co-founded by the European Union under the project “Collaborative learning and innovative teaching in brain drug screening” (2023-1-PL01-KA220-HED-000160284). It is licensed under a Creative Commons Attribution-NonCommercial (CC BY-NC) license, allowing sharing and adaptation for non-commercial purposes with proper attribution.
Send a message
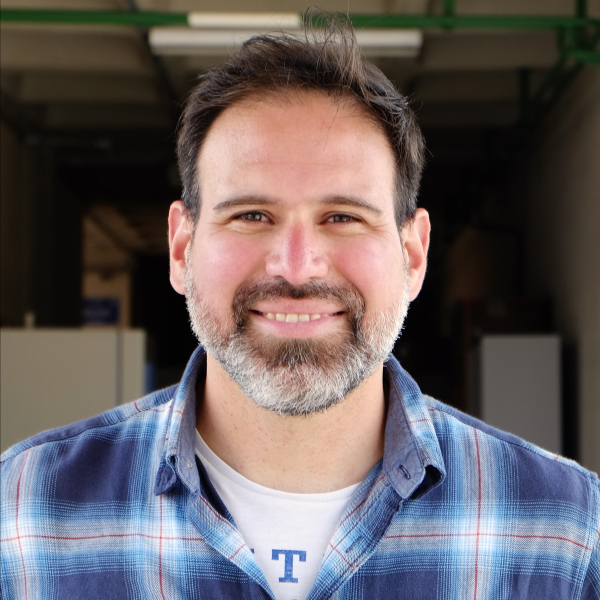
Adding comments is only possible for registered users.
Sign in