Examining brain networks using large-scale electrophysiological recordings and high-resolution optogenetics
Large-scale electrophysiological recordings combined with high-resolution optogenetics enable precise monitoring and manipulation of neural activity with unparalleled spatiotemporal accuracy. Dr. Manuel Valero expertly utilizes these techniques, advancing our understanding of brain networks and their role in behavior and neurological disorders.
This video is licensed under a Creative Commons Attribution-NonCommercial-NoDerivatives (CC BY-NC-ND) license. You are free to share it for non-commercial purposes with proper attribution, but no modifications or adaptations are allowed.
1. Why use this method?
Large-scale electrophysiological recording is an indispensable tool in neuroscience, enabling the measurement of individual neuronal activity with high temporal resolution in different brain regions in vivo. At the same time, optogenetics has become the gold standard in the field, allowing rapid and targeted manipulation of genetically defined neuron types. By expressing light-sensitive proteins in specific neuronal populations, this method facilitates the identification of distinct neuronal subtypes. Combined, large-scale neuronal recording and optogenetic stimulation provide a powerful approach to dissecting the neural circuits underlying behavior, cognition, and the neuropathology of neurological disorders. The novel approach to this method involves the use of microscopic light-emitting diodes (μLEDs) integrated with recording sites on the silicon neural probes, providing spatiotemporal precision for parallel stimulation and recording.
2. What you’ll need
1. Stereotaxic surgery –μLED probe implantation
- Transgenic mice/rats expressing channel rhodopsin on targeted neurons,
- Stereotaxic apparatus and anesthesia system,
- Trephine
- μLED probe
- Micromanipulator,
- Ground and reference wires,
- Microdrive,
2. In vivo recordings
- Electrophysiology system (e.g. Intan RHD2000 system),
- Analog-digital converter,
- Signal amplifier,
- Lightweight multi-strand Litz wires
- Waveform generator,
- Current generator (e.g., OSC1Lite, NeuroNex Michigan Hub).
3.Data management and analysis
- Spike sorting software (e.g., KiloSort),
- Scripts (MATLAB, Python) that allow the data analysis,
3. Step-by-step instructions
1.Stereotaxic surgery –μLED probe implantation
- Put the animal under anesthesia and perform a bilateral craniotomy using selected coordinates.
- Implant the ground and reverence wires (e.g., in the skull and cerebellum, respectively).
- Implant the μLED probe using a micromanipulator, lowering it deeper into the brain tissue.
- Mount a probe on a microdrive.
- Starting the day following implant, gradually lower the probe drive down to the target region by turning the screw on the microdrive.
2. In vivo recording
- Use lightweight multi-strand Litz wires to connect aμLED probe with a voltage source.
- Using an electrophysiology system, record the spontaneous spiking activity.
- Perform the photostimulation by applying an adequate voltage using a waveform generator.
- Apply 15 pulses of stimulation (over a time period equal to more than 3s), followed by recording the baseline activity).
3. Data management and analysis
- Use Kilosort to perform a spike sorting.
- Develop and/or use scripts that allow to analyze the electrophysiological data.
4. Practical tips
- Prior to the in vivo recordings, a μLED probe must be attached to a metal or plastic drive connected to the plastic stereotaxic holder, which will allow for post-implant depth adjustment of the probe. While is an standard approach for fibers, this is not possible for μLEDs.
- A silicon probe microdrive can be purchased. However, it is possible to build it from scratch.
5. Critical appraisal & implications for future research
The development of μLED probes in neuroscience presents both significant challenges and promising opportunities. A primary challenge lies in the limitations of internal quantum efficiency (IQE) and photon extraction efficiency, which limit the effective conversion of electrical power into light. Despite these limitations, μLEDs can still activate neurons without causing significant tissue heating, making them viable for precise neural stimulation.
Another critical challenge is the requirement for advanced data analysis methods to interpret the complex signals generated during experiments. This complexity underscores the need for continued refinement of both hardware and software to exploit the potential of μLED technology fully.
Looking towards the future, μLED probes represent a transformative frontier in neuroscience, offering unprecedented opportunities for precise manipulation of neural circuits. With advances in fabrication and integration, these probes could enable highly targeted neural stimulation with improved spatial resolution, opening new avenues for studying brain function and behavior. The potential to independently control different neuronal populations or inputs within deep brain structures highlights the future role of μLEDs as a powerful tool in neuroscience research.
This protocol is part of the Braining project, co-founded by the European Union under the project “Collaborative learning and innovative teaching in brain drug screening” (2023-1-PL01-KA220-HED-000160284). It is licensed under a Creative Commons Attribution-NonCommercial (CC BY-NC) license, allowing sharing and adaptation for non-commercial purposes with proper attribution.
Send a message
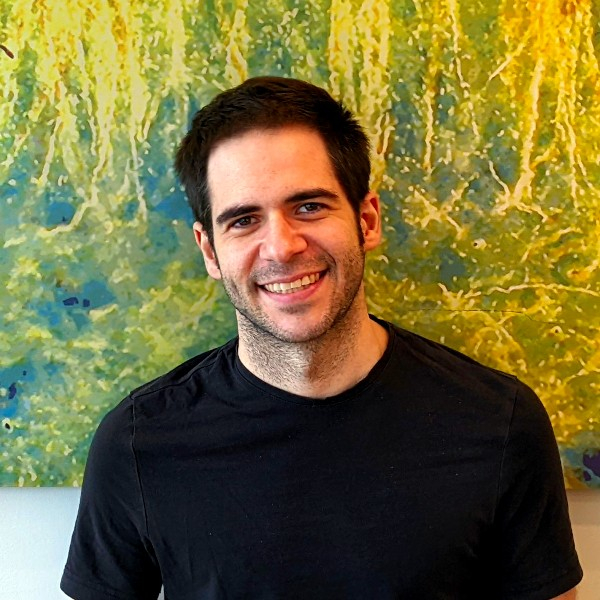
Adding comments is only possible for registered users.
Sign in